In Part One of this series, we discussed the care and feeding of corals’ symbiotic dinoflagellates (Symbiodinium species, or zooxanthellae.) To recap, corals (as are all living things) are composed of proteins, carbohydrates, lipids, and ash (non-volatile, inorganic substances such as metals.) The coral animal obtains some of the organic substances through feeding (heterotrophy) or translocation from zooxanthellae (which are autotrophic.) Hence corals obtain sustenance from various sources in a process called mixotrophy, a combination of heterotrophy and autotrophy.
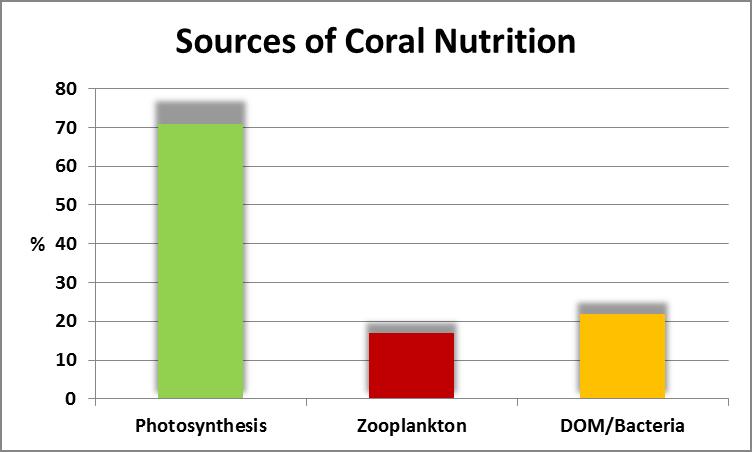
Figure 1. Photosynthesis can provide most of the carbon requirements for coral tissue growth. Other important sources are plankton (zooplankton and phytoplankton) as well as DOM (dissolved organic material) and bacteria.
In order for mixotrophy to work properly, light intensity (for the zooxanthellae) and water motion (primarily for the coral animal to feed and absorb nutriment through a diminished Momentum Boundary Layer, with zooxanthellae being indirectly affected) must be within upper and lower limits. The importance of this cannot be overstated. Generally, photosaturation (where the rate of photosynthesis is no longer proportional to light intensity) occurs for many corals at light levels less than 400 µmol∙m²∙sec (or about ~20,000 lux.) See Part One for details: http://www.advancedaquarist.com/2014/11/aafeature
As a quick review, Figure 1 gives us a rough idea of nutritional sources and their proportions for photosynthetic corals.
Water motion deserves a bit more examination. Research has shown that sessile invertebrates’ feeding rates are related to water velocity. If insufficient prey is encountered, the coral, in order to conserve energy, might withdraw its polyps. At the other end of the spectrum, polyps will be withdrawn if water velocity is too high and danger of damage occurring is possible. Table 1 shows optimal water velocity rates for various invertebrates.
Coral | Velocity (inches/sec) |
---|---|
Acanthogorgia vegae | 3.1 |
Agaricia agaricites | 7.1 |
Agaricia agaricites (bifacial) | 11.8 |
Agaricia agaricites (horizontal) | 7.1 to 19.7 |
Briareum asbestinum | 2.3 to 4.7 |
Dendronephthya hemprichii | 3.9 to 9.8 |
Eunicea tournefortis | 2.4 to 4.72 |
Galaxea fascicularis | 3.9 |
Lophelia petusa | 1 |
Melithaea ochracea | 3.1 |
Plexaura dichotoma | 2.4 to 4.72 |
Porites porites | 3.5 to 4.33 |
Pseudopterogorgia americana | 2.4 to 4.72 |
Subergorgia suberasa | 3.1 |
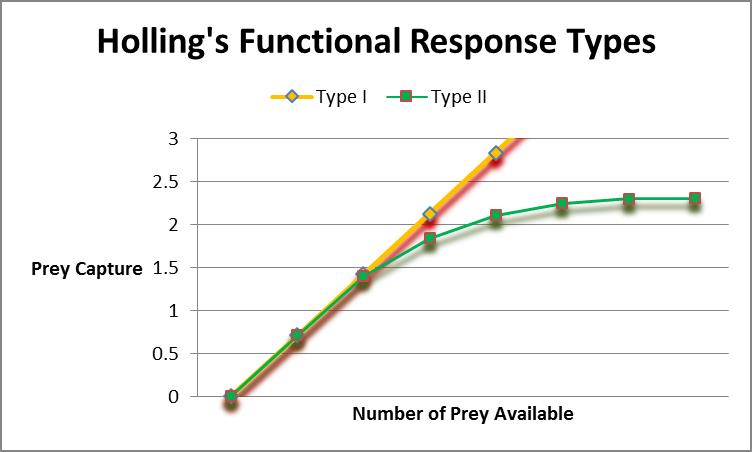
Figure 2. Corals’ feeding responses are dependent upon water motion and number of prey. Holling’s Functional Responses describe the latter.
Related to water velocity/feeding rates is a concept called Functional Response. This idea was developed by ecologist C.S. Holling and examines the relationship between prey density and the rate at which prey is eaten. Holling’s Type I describes a relationship where the consumer’s ingestion of prey and prey density is linear. In other words, the time to digest food is considered negligible and eating doesn’t interfere with hunting (or in this case, capture of prey.) Holling’s Type II refers to situations where the consumer’s ‘attack time’ (or perhaps more accurately ‘encounter time’ in cases of corals) is limited by ‘handling time’ (‘digestion time’.) A Type III exists, but involves situations where the predator learns and adapts, or switches prey (the former is an interesting concept that might pertain to aquarium corals. There are reports that some corals have been ‘trained’ to open polyps for prey capture at certain times of the day, without tactile or chemical stimuli.) Figure 2 charts demonstrates relationships of Type I and II functional responses.
Anthony (1999) found the stony coral Porites cylindrica conformed to Holling’s Type II, with particle capture peaking at relatively low concentrations of ‘prey’. On the other hand, several SPS coral genera (Pocillopora damicornis, Montipora digitata, and Acropora millepora) showed feeding responses that were linear to particle concentration (Type I response at concentrations of 1 to 30 mg/l.) However, assimilation by the corals was inversely proportional to concentration. With that said, Anthony reported suspended matter could cover up to ½ of carbon requirements and 1/3 of the nitrogen.
The Smaller the Mouth, The Smaller the Prey
This is a simple concept yet one commonly ignored when we examine particle size of some commercially available coral foods. We will examine the size of particles ingested by small polyped stony corals in this article. There has been relatively little research conducted on single- or large-polyp stony corals.
Micrometers
A micrometer is 1/1,000,000th of a meter. To put this in perspective, a fine human is as little as 17 micrometers in diameter. We’ll use micrometers (abbreviated as µm) as the standard for describing size of coral prey organisms.
Particulate prey include a number of animate and inanimate objects ranging in size from the size of bacteria (perhaps 0.5 micrometers) to 200 micrometers and even larger.
This month, we’ll examine various food sources for the coral animal. These involve organic and inorganic substances, both particulate and dissolved. But first:
Predation of Cyanobacteria, Bacteria, and Algae by Symbiodinium spp. (Zooxanthellae)!
Before continuing our study of coral nutrition, we’ll begin by examining the consumption of cyanobacteria, bacteria, and algae by zooxanthellae. Cyanobacteria, or cyanophyta, are a group of photosynthetic bacteria. They are sometimes called blue-green algae due to their color (cyan = blue.)
Synechococcusspp. are small (0.6 to1.5 microns) cyanobacteria that inhabit shallow-water marine environments (although some freshwater species have been described.) They are plentiful and can number from 50 to as many as 4,000 per drop (assuming 20 drops per milliliter.) There is some reason to believe some species could ‘fix’ nitrogen gas and convert it into organic nitrogen compounds. Their main photopigment is chlorophyll a, but they also contain other pigments, including phycoerythrin (a red, light-harvesting photopigment.) See Figure 3.
Jeong et al. (2012) found free-living Symbiodinium, as well as symbiotic zooxanthellae (acquired from the stony coral Alveopora japonica), have the ability to feed on heterotrophic bacteria, cyanobacteria (Synechococcusspp.), and microalgae (through a process known as phagocytosis.) Free-living dinoflagellates did not grow when denied a these nitrogen sources, but grew when fed with any of these prey. This discovery alters the concepts of how dinoflagellates thrive in nutrient-depleted environments.
How Corals Feed
Corals have evolved a number of ways to feed – their food can be dissolved and absorbed through their ectoderm (skin), by capturing particles in a mucus net, creating currents around their mouths with cilia-like structures, or harpooning or entangling prey with cnidocytes and nematocysts. Nematocysts ‘fire’ by either tactile or chemical stimuli.
Some coral species feed by tentacular capture only (such species found Poritidae and Pocilloporidae), while others feed by ‘suspension capture’ (or culture) of bacteria and other fine particulates in a mucus net. Others use both strategies.
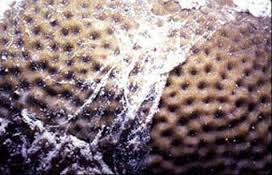
Figure 4. A mucus net partially covers this corals. It acts as a trap for particulates and a food source for bacteria.
Mucus Net
Some corals cast a net or network of filaments composed of internally-generated mucus in order to capture prey. In a study of Atlantic corals, Lewis and Price (1975) found corals within the Family of Agariciidae secreted mucus from the epidermis of the oral disk. Hence, corals with retracted polyps, either during the day or night, could continue to capture prey by acting as mucus broadcasters, suspension feeders, and predatory feeding on plankton. See Figure 4.
Note: Corals of Family Agariciidae are found in the Atlantic and Pacific and include genera Agaricia, Coeloseris, Gardineroseris, Helioseris, Leptoseris, Pachyseris, and Pavona. Mucus nets are also seen with Fungia and some other corals of Family Fungiidae. Mucus coatings are common among many soft and stony corals, especially those of genera Sarcophyton and Porites.
Bacteria and the Mucus Net
Coral mucus consists mostly of excess carbon fixed by photosynthesis or obtained heterotrophically. As Figure 5 shows, mucus discharged by at least some corals contains lipids (fats) and carbohydrate (glucose, a simple sugar.) Both are energy rich and excellent food sources for bacteria. Hence, a corals’ mucus net could be expected to contain bacteria as well as particulate matter.
Nematocysts and Cnidocytes – Nature’s Harpoons
Cnidarians have internal structures called cnidocytes which contain natural harpoons called nematocysts. See Figure 6.
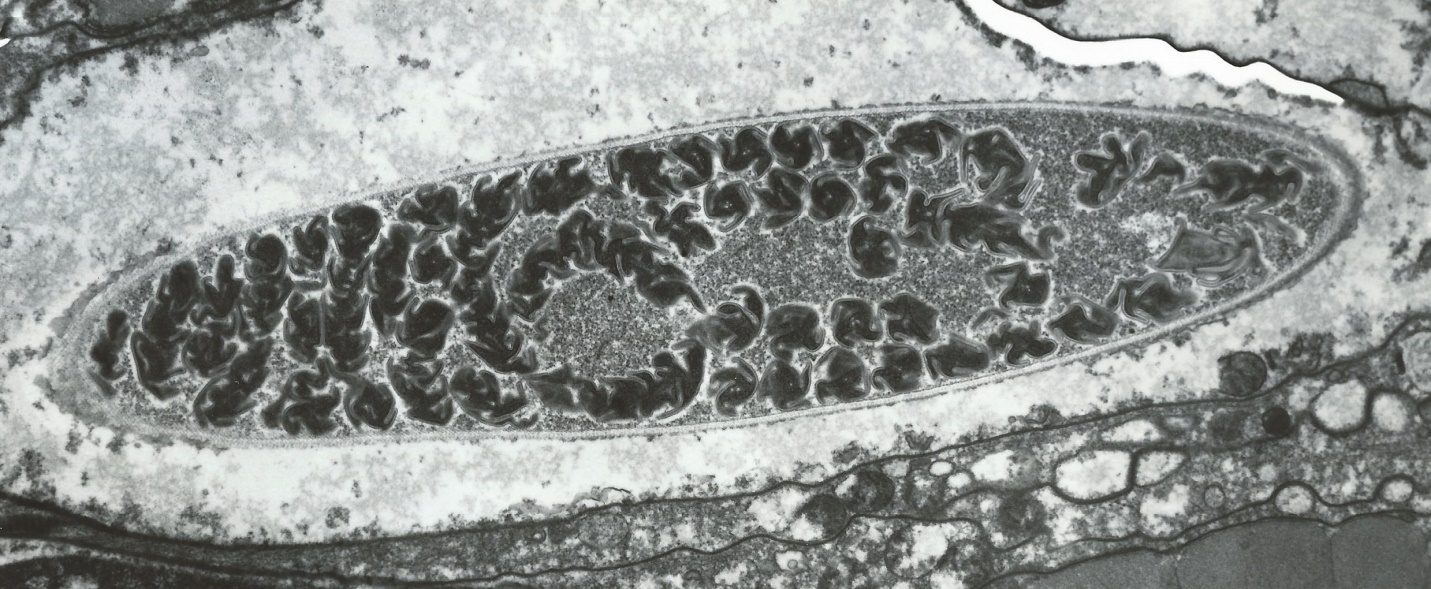
Figure 6. A cnidocyte – corals’ structures for prey capture. Note the coiled nematocyst (a natural harpoon) within the organelle. A photomicrograph taken with an electron microscope at a power of 15,900x. Courtesy of Drs. Denny Aaron and Steven Poet, University of Georgia.
When a ‘trigger’ on a coral’s surface is touched, a ‘door’ called an operculum springs open and the thread-like nematocyst is discharged. The nematocyst is loaded with venom that can immobilize relatively large prey. These toxic threads can also be used as defensive and offensive mechanisms. Nematocysts can also simply entangle and trap prey. See Figure 7.
Bacteria
Bacteria (from the Greek word for ‘staff cane’ since the first discovered resembled rods) are a diverse group of prokaryotic micro-organisms and are thought to be the first organisms on earth. They usually range in size from 0.5 to 5 micrometers in length although a few are much larger and can be visible to the unaided human eye.
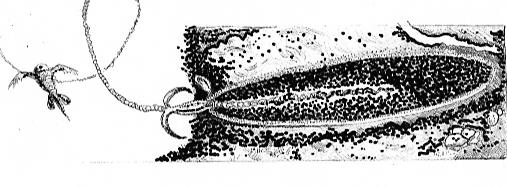
Figure 7. What the cnidocyte in Figure X might look like with its nematocyst discharged and entangling prey.
Nature is the great recycler of substances and bacteria make this possible. Aquarists are likely most aware of bacteria that cycle nitrogenous compounds (such as the conversion of ammonia to nitrite and nitrate), although those converting carbon, phosphorus, and others are also present.
Bacteria levels in an aquarium can be high or low depending upon water quality management techniques (Feldman et al., 2011.) It seems that tanks with ‘aggressive’ water management protocols have bacterial numbers less than seen in some marine environments, while passive maintenance (no skimmer, occassional water changes) results in higher bacteria counts.
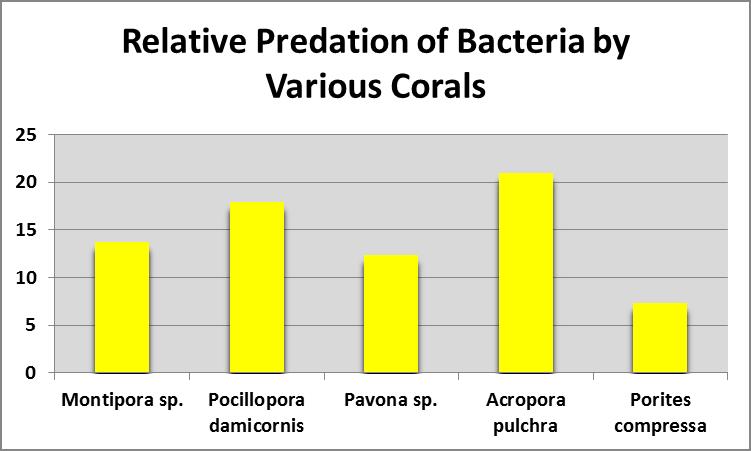
Figure 8. All small-polyp stony corals examined fed on bacteria. From Sorokin, 1973.
Sorokin (1973) fed corals with bacterioplankton and found all small-polyp stony corals examined rates of ingestion and assimilation of bacteria. See Figure 8.
Interstitial pores within the coral skeleton can harbor a number of bacteria taxa.
Predation of Green Algae by Corals
Many consider corals to be ravenous predators of zooplankton, but at least some corals ingest green algae as well. Sorokin (1973) showed that the green alga Platymonas was ingested by small-polyp corals, including Acropora, Montipora, Porites, Pocillopora and Pavona species. Platymonas is often (and easily) cultured by hobbyists as a food for rotifers (Brachionus spp.) and brine shrimp (Artemia spp.) Platymonas cells are approximately 15 micrometers in diameter. See Figures 9 and 10.
Predation of Dinoflagellates by Corals: Amphidinium and Gymnodinium
It is ironic that an animal (coral) dependent upon one dinoflagellate genus (Symbiodinium) would ingest and digest other types of dinoflagellates. Sorokin (1973) found that corals could eat the dinoflagellate genera Amphidinium and Gymnodinium. These dinoflagellates are about 35 – 60 micrometers in diameter. (Symbiodinium are 6 to 13 micrometers in diameter, depending upon clade or species.) See Figures 11 and 12.
As Figure 13 shows, ingestion and digestion of dinoflagellates by corals appears to be species specific with a Pocillopora species ingesting most, while Porites compressa ate none.
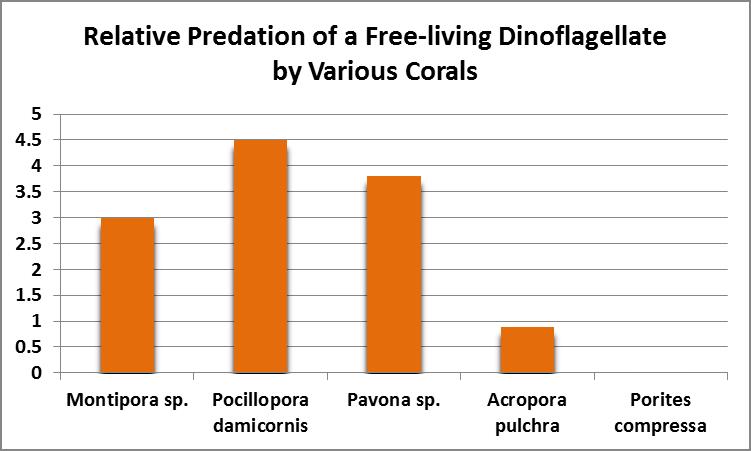
Figure 13. Dinoflagellates were eaten by all corals tested except for Porites compressa. From Sorokin, 1973.
Predation of Ciliates by Corals
Ciliates are a group of protozoans (from the Greek word ‘protozoon’ where protos = first and zoon = animal) characterized by hair-like structures called cilia. Sorokin (1973) found that corals will ingest the ciliate Euplotes (which are 80-200 micrometers in length.) This ciliate is photosynthetic and contains zoochlorellae (a symbiotic green algae.) See Figure 14.
Brine Shrimp (Artemia spp.)
Brine shrimp have been a staple of captive fish and invertebrate culture for decades. It is estimated that 2000 tons of brine shrimp cysts are marketed annually. Their ability to live in brine lakes allows them to grow in tremendous numbers without threat of being eaten by fishes. In addition, their egg cysts can be successfully stored dry for years. Freshly-hatched brine shrimp (nauplii) are ~400 µm in length and have been used in a number of coral feeding experiments. See Figure 15.
Coprophagy: The Scoop on Poop
Coprophagy, or eating of feces, is not a subject commonly discussed in polite company (or impolite company for that matter.) However, it is common in the bacterial world. It is also seen among some reef fish species. Feces can combine with other substances and form ‘marine snow.’
A concise investigation of nutritive value of fish poop was conducted for this article. Information on particle size, and nutrient content (total nitrogen and total phosphorus) will be shown below.
First and foremost, can the particle size be small enough for ingestion by small polyped corals? Samples of fish faeces (from Yellow Tangs (Zebrasoma flavescens) and a Chevron Tang (Ctenochaetus hawaiiensis) were drawn from an aquarium with no substrate (‘bare bottom.’) These were placed in a 1-liter bottle, capped, and shaken vigorously to simulate maceration by a pump impeller or propeller. The resulting suspension was sequentially filtered through filters with pore sizes of 1.5, 1.0, and 0.45 micrometers. Results are shown in Figure 16.
As Figure 16 demonstrates, particle size can be small enough to feed any small-polyp stony coral. The second question asks about organic content. To this end, a suspension was filtered through a pre-combusted (and pre-weighed) glass fiber filter with a pore size of 1.5 micrometers. The filter, with captured solids, was dried at 103°C for one hour, cooled in a desiccator, and weighed with an analytical balance. Weight of the dry suspended material within the sample was determined using this formula:
[(a-c) / volume of sample (milliliters)] x 1,000
Once suspended solid content was determined, the filter (with retained material) was placed in a muffle furnace (temperature of 550°C) and volatized for 20 minutes. The volatile content (generally considered to be the organic portion) was determined with this formula:
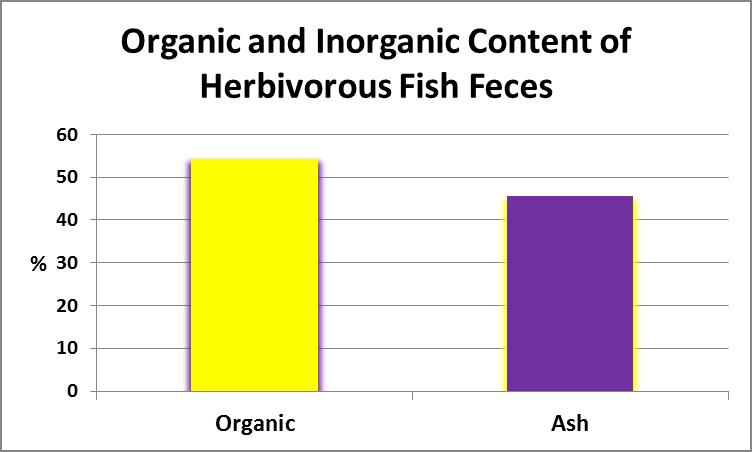
Figure 17. The inorganic, or ash, content of this sample was almost ½ of the total content by weight.
[(a-b) / volume of sample (milliliters)] x 1,000
where a is filter and sample dry weight; b is weight of volatized filter and sample, and c is dry filter weight. See Figure 17.
We have determined macerated fish waste is potentially of the correct size for ingestion by small polyp stony corals and that it has substantial organic content. The next examinations were for Total Nitrogen and Reactive, Condensed and Total Phosphorus.
A dearth of nitrogen can limit growth in any organism, hence the Total Nitrogen content of fish waste was determined. This procedure involves chemical oxidation of the sample with heat acting as a catalyst. Figure 18 shows the result.
According to Liebig’s Law of the Minimum, plant growth (including zooxanthellae) will be poor if one necessary nutrient is absent even if all others are in plentiful supply. Hence, Reactive phosphorus (orthophosphate) is often targeted for removal as it promotes algae growth and is easily removed chemically. It is possible to take orthophosphate to levels undetectable by sophisticated instrumens. However, particulates suspended in the water column can contain phosphorus. Total Phosphorus content was determined by oxidation with persulfate and applied heat. Acid Hydrolyzable Phosphorus was determined by conversion to orthophosphate through use of acid and heat. Orthophosphate was tested colorimetrically (the method used by most hobbyists. See further clarification of these types of phosphorus below.) The results are shown in Figure 19.
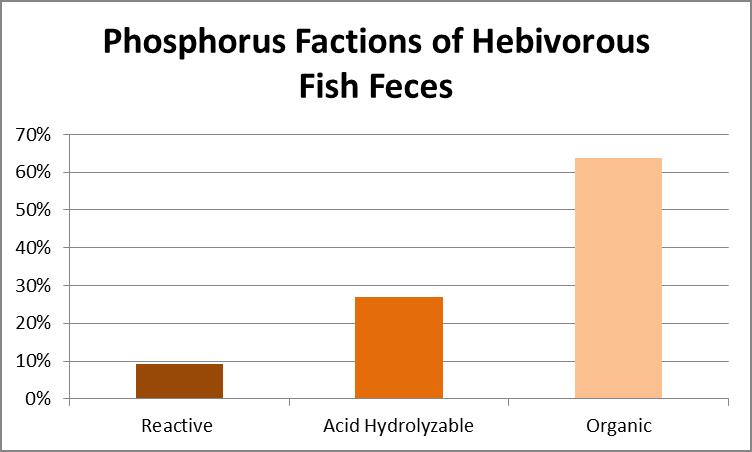
Figure 19. Phosphorus content. Organic phosphorus is at about 60%, condensed phosphorus is just less than 30%, while reactive P is almost 10%.
- ReactivePhosphateor orthophosphate: Reactive P (also called ortho-phosphate) is ‘bio-available’ for promotion of algae growth. Reactive P can be dissolved or suspended. Colorimetric tests forphosphates commonly available to hobbyists measure only orthophosphates although small amounts of easily hydrolysable inorganic and organic P might be included in the result. The most abundant forms of orthophosphates occurring between pH 5 to 9 in aquatic environments are HPO4-2, H2PO4–, PO4-3, HPO4-2, and H2PO4(Stumm and Morgan, 1981).
- Acid-hydrolyzable (A-H) Phosphate or CondensedPhosphate: Acid-hydrolyzable P can be found as dissolved or suspended forms. It may be in pyro-, meta-, tripoly-, and other forms of polyphosphates (such as hexametaphosphate). The term ‘acid-hydrolyzable’ is preferred over ‘condensed’. Phosphorus in the sample as measured by the sulfuric acid hydrolysis procedure, then pre-determined orthophosphates are subtracted (EPA, 1979). The acid-hydrolysis method reports dissolved and particulate condensedphosphatethat is converted to dissolved orthophosphate through acidification of the sample (APHA, 1998). It is referred to as Dissolved Hydrolyzable P or Total Hydrolyzable P, when filtered or unfiltered samples are tested respectively.
- Total Phosphorus: The sum of organic and inorganic forms of phosphorus. As with reactive and condensed P, Total P can either dissolved or suspended. It is determined colorimetrically only after a severe oxidative digestion process where Total P is converted to orthophosphate
Size of Particulates in Aquarium Water
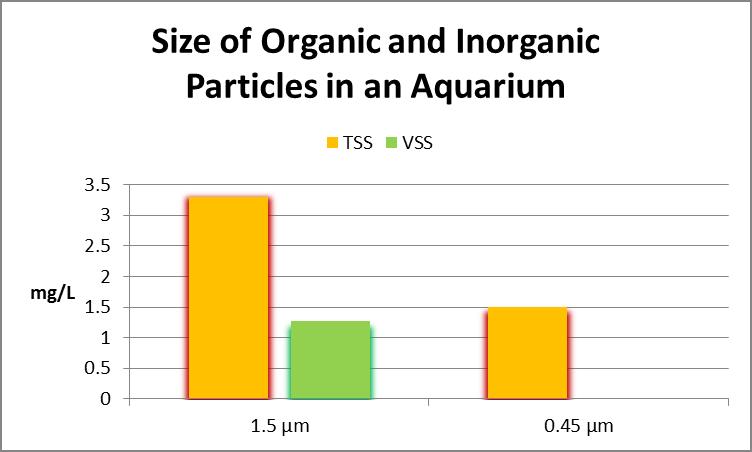
Figure 20. Aquarium water particulate matter. This aquarium contained ~1.3 mg/L of organic material with a particle size of at least 1.5 µm. Since the filter used for determining smaller particulate matter was made of cellulose, it was not possible to determine organic content.
Water clarity is an important aesthetic issue in an aquarium; hence mechanical filtration is often employed to this end. Hobbyists might be surprised to learn that, even with mechanical (micron cartridges, filter socks) there is still a considerable amount of material suspended in the aquarium water.
Testing of water from a biologically under loaded aquarium showed the water contained over 3 mg/L of particles with a diameter of at least 1.5 µm (slightly less than half of this material was organic in nature.) A filter with a pore size of 0.45 µm retained 1.5 mg/L suspended solids. This aquarium utilizes a filter sock with a pore size of 200 micrometers for mechanical filtration. See Figure 20.
Marine Snow
Marine Snow is a term for conglomerations of suspended organic matter. On coral reefs, marine snow can be composed of remains of dead organisms including animals, plants and algae, coral mucus (which harbors bacteria), fecal matter, and other material.
Dissolved Food Sources
To begin our discussion of dissolved substances, we should define the word ‘dissolved.’ Generally, a substance deemed to be dissolved is one that will pass through a filter with a very small pore size (often 0.2 – 0.45 micrometer in diameter.)
There are many categories of dissolved food sources. Some contain carbon, while others contain nitrogen or phosphorus, combinations of these, or any of many others.
An examination of metal uptake for use in skeleton formation will be the subject of a separate article.
Absorption
From the Latin word absorbere, meaning ‘to suck in, swallow,’ absorption of dissolved substances is an important process in coral nutrition. Water motion must be sufficient to minimize the momentum boundary layer (or MBL – a ‘coating’ of stagnant water that surrounds the coral, or any other aquatic animal for that matter.) If water velocity does not minimize the MBL, concentration gradients may exist and these could potentially rob the animal/symbionts of necessary nutriment.
Dissolved Organic Material (DOM)
Dissolved Organic Material (DOM) is a broad classification of mainly carbon-based compounds found in water. Coastal seawater contains 3-6 mg/l organic carbon (Chailow, 1971.) Coral researchers have used various compounds in DOM-uptake experiments including algal extracts, dissolved amino acids, and sugars.
Sorokin (1973) examined the uptake of DOM by various corals. This particular compound was hydrolyzate of algal protein (various amino acids produced by ‘splitting’ algal proteins with an acid, followed by neutralization of acidic pH. Hence, this DOM contains carbon, nitrogen, and relatively minor amounts of phosphate and others.)
Dissolved Organic Carbon (DOC)
Dissolved Organic Carbon is a broad category of organic substances found in aquatic environments. DOC usually is a result of decaying animal or plant matter, although it can be the result of materials leached by marine plants and algae. Some DOC compounds (sugars, for example) are said to be labile, meaning they are easily consumed by bacteria. Others are refractory and are not easily used by bacteria.
Dissolved Inorganic Carbon (DIC) can exist in the forms of carbon dioxide, carbonic acid, carbonates and bicarbonates.
Total Organic Carbon
Total Organic Carbon (TOC) is the sum of particulate and dissolved organic carbon. Aquarium water contains up to 1.5 mg/L TOC (Feldman et al., 2009) if protein skimming is used. It presumably could be higher if no aggressive means of water quality management are used.
Primary Production
‘Primary production’ refers to the manufacture of organic compounds by plants and algae.
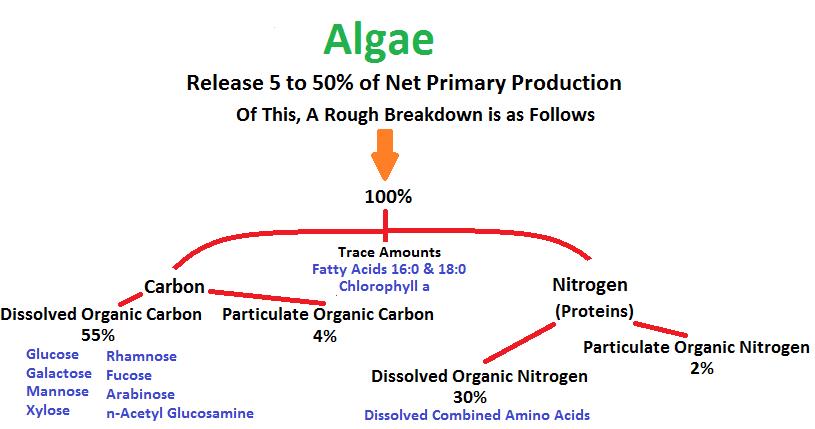
Figure 21. Caulerpa and Halimeda algae can release a number of compounds corals can utilize as food.
As Figure 21 shows, dissolved organic carbon in the form of sugars is by far the major sort of organic matter released, followed by dissolved combined amino acids.
These are some of the sugars produced and released to the surrounding water:
- Glucose (C6H12O6) is a simple sugar and an important product of photosynthesis. Glucose is used by cells as an energy source and is also a precursor to amino acids and fatty acids.
- Galactose (C6H12O6) : From the Greek word galakt or milk, it is a simple sugar and is an epimer of glucose (that is, the chemical formula of galactose is the same as that as glucose, but its structure is slightly different.)
- Mannose (C6H12O6) is also an epimer of glucose.
- Xylose (C5H10O5): First isolated from wood, xylose’s name originates from the Greek word xylon, or wood.
- Arabinose (C5H10O5) found in pectin (from cell walls in terrestrial plants and often used as a gelling agent) and hemicellulose (also found in plant cell walls.)
- Rhamnose (C6H12O5)
- Fucose (C6H12O5)
- Sucrose (C5H10O5) an epimer of arabinose
- Ribose (C5H10O5)
Release of Organic Carbon by Marine Algae
It is well known that zooxanthellae within coral tissues are ‘leaky’ and provide some of their photosynthetic products. The same applies to micro- and macro-algae growing within the aquarium.
Carbohydrates Released by Caulerpa and Halimeda Algae
Figures 21 and 22 examine the release of organic material (in the form of carbohydrates) by algae commonly maintained in aquaria – Caulerpa and the calcifying Halimeda.
As Figure 22 demonstrates, algae commonly found in marine aquaria can release a number of sugars, so it would seem there would be a steady supply of carbohydrates. Or is there? Stephens (1962) examined sugar uptake by the solitary stony coral Fungia scutaria and found, at least for this coral, that glucose is the most preferred compound. See Figure 23.
Dissolved Inorganic Nitrogen
Dissolved Inorganic Nitrogen is defined as the sum of ammonia/ammonium, nitrite, nitrate, and nitrogen gas.
Since nitrogen gas composes ~79% of earth’s atmosphere, it is only natural that seawater contains dissolved nitrogen gas. Nitrogen gas is not readily bio-available to organisms except for a group of bacteria that possess the ability to ‘fix’ this gas. These bacteria generally have a symbiotic relationship with a number of plants (such as peanut plants, alfalfa, and others.) When these cyanobacteria die (or are eaten) the nitrogen within their tissues is available as fertilizer.
Lesser et al. (2004) found symbiotic nitrogen-fixing cyanobacteria in the Caribbean stony coral Montastrea cavernosa. Interestingly, the reddish-orange color of the coral is due to the presence of phycoerythrin within the bacteria. When the bacteria die and decompose within the tissues of the coral, they potentially provide a source of nitrogen. I have some reason to believe these cyanobacteria also exist in some (not all) orange Montipora digitata specimens. As discussed earlier in this article, Jeong et al. (2012) found Symbiodinium acquired from the stony coral Alveopora japonica can feed on Synechococcusspp., cyanobacteria thought to fix nitrogen gas. It is an interesting thought that some corals could host a symbiont capable of supplying organic nitrogen manufactured from dissolved nitrogen gas.
Uptake of Inorganic Nitrogen: Ammonia and Nitrates
As organic nitrogen compounds degrade, they become ammonia and eventually nitrates through actions of aerobic bacteria. Ammonia and nitrate can be absorbed by at least some corals. See Figure 24 (which demonstrates that ammonia/ammonium is the preferred source of inorganic nitrogen.)
Tests have shown that ammonia spikes occur just after feeding fishes in an aquarium.
Dissolved Organic Nitrogen
Dissolved Organic Nitrogen can exist in many forms – as urea, dissolved free amino acids, combined amino acids, and so on.
Urea
Produced by mammals and some marine fishes, urea (CH4N2O) is a major end product of nitrogen metabolism. The stony coral Stylophora pistillata can absorb urea and other organic nitrogen sources. See Figure 25.
Urea eventually degrades to ammonia and becomes a source of inorganic nitrogen.
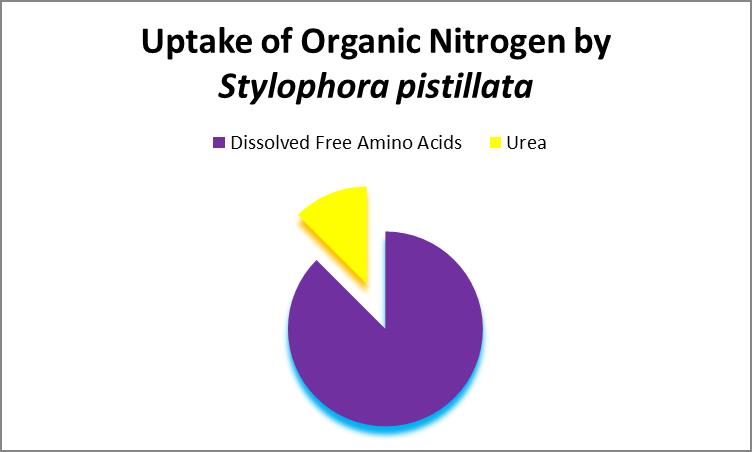
Figure 25. Dissolved organic nitrogen includes urea, free and combined amino acids, among others. Grover et al., 2008.
This researcher also found that the stony coral Stylophora pistillata can absorb dissolved free amino acids. Note: A future article will be devoted to the importance of amino acids in coral nutrition.
Dissolved Inorganic Phosphorus (DIP)
Dissolved inorganic phosphorus generally refers to ortho-phosphate (or reactive phosphate.) Ortho-phosphate is the sort of nutrient that stimulates plant and/or algal growths. While it is tempting to think that ortho-phosphate could be a primary source of phosphorus in nutrient-scarce waters, it is not. Sorokin (1973) found that many corals popular in the aquarium trade (Acropora, Montipora, Pocillopora, etc.) actually obtain more phosphorus by ingesting bacteria that have organic phosphorus bound in their cellular walls.
In Closing
We have examined corals’ uptake of various dissolved substances (carbohydrates, inorganic and organic phosphorus, as well as inorganic and organic forms of nitrogen.)
- At least some Symbiodinium species can ingest and assimilate bacteria, cyanobacteria, and algae.
- Corals can capture particulate food particles by use of stinging cells or mucus net.
- Prey capture can be a function of concentration and water movement.
- Small polyp stony corals can ingest particles ranging in size from 0.45 µm to ~400 µm and perhaps larger (see Figure 26.)
- SPS corals can ingest and assimilate bacteria, green algae, dinoflagellates, ciliates, and larger zooplankton.
- Organic phosphorus (bound in bacteria tissue) is the preferred phosphate source (as opposed to dissolved reactive phosphate.)
- Some algae often grown in aquaria (Halimeda and Caulerpa) release carbohydrates (sugar) that be absorbed by corals. Glucose is the preferred sugar of some Fungia specimens.
- Ammonium is preferentially absorbed by corals. Nitrate is also absorbed.
- Fish poop is a potential source of nitrogen and phosphorus.
- Urea (from fishes, and a source of nitrogen) can be absorbed.
- Even well-maintained aquaria (protein skimmers, filter socks) can contain suspended organic matter.
- Some corals are host to nitrogen-fixing bacteria. These are a source of nutriment when they die (or are consumed.)
- Amino acids (either combined or free) can be a nitrogen source.
Amino Acids
Production of amino acids by zooxanthellae and corals as well as absorption of free amino acids and ingestion of nitrogen-containing compounds is a complex subject and deserves separate examination. Hence, our discussion for this time concludes. Next time we’ll discuss amino acids. I’ll also present some rather interesting results of tests on a commercially available amino acid supplement.
References
- Ferrier, M., 1991. Net uptake of dissolved free amino acids by four scleractinian corals. Coral Reefs, 10: 183-187.
- Grover, R., J-F. Maguer, D. Allemand, and C. Ferrier-Pages, 2008. Uptake of dissolved free amino acids by the scleractinian coral Stylophora pistillata. J. Exp. Biol., 211: 860-865.
- Feldman, K., A. Place, S. Joshi, and G. White, 2011. Bacterial counts in reef aquarium water: Baseline values and modulation by carbon dosing, protein skimming, and granular activated carbon filtration. http://www.advancedaquarist.com/2011/3/aafeature
- Lewis, J. and W. Price, 1975. Feeding mechanisms and feeding strategies of Atlantic reef corals. J. Zoology, 176(4):527-544.
- Lesser, M., C. Mazel, M. Gorbunov, and P. Falkowski, 2004. Discovery of symbiotic nitrogen-fixing cyanobacteria in corals. Science
- Anthony, K., 1999. Coral suspension feeding on fine particulate matter. J. Exp. Mar. Biol. Ecol., 232(1): 85-106.
- Sorokin, Y., 1973. On the feeding of some scleractinian corals with bacteria and dissolved organic matter. Limnol. Oceanogr., 18:380-385.
- Stevens, G.C., 1962. Uptake of glucose from solution by the solitary coral Fungia. Science 131:1532-1534.
- Sommerville, K. and T. Preston, 2001.Characterization of dissolved combined amino acids in marine waters. Rapid Comm. Mass Spectrom., 15(15): 1287-1290.
- Wijgerde, T., 2013. Coral Feeding: An Overview. http://www.advancedaquarist.com/2013/12/aafeature
Fish Poop, Algae “Poop”, Sponges + Light = Balanced Coral diet
…so just feed the fish a balanced diet and the fish/Algae/Sponges feed the corals.
I assume all those “floral snow” dry products are a waste of time.